Design to survive
June 23, 1997
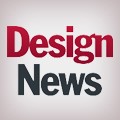
An underwater explosion, repeated jolts from a 100,000-lb excavator, a helicopter crash, 30-ft drops to a steel surface. While these may sound like stunts from the latest Hollywood action film, they're actually a short list of real situations presented to engineers who had to design products to survive them.
No special effects would do. For assistance, each turned to a tool that was at one time the province of engineers bearing a masters degree or Ph.D.--finite element analysis (FEA).
Extreme environments lend themselves well to simulation. While computer modeling certainly presents its own challenges, recreating actual bangs, bashes, strains, and bombs in controlled tests--and capturing data from them--can be terribly expensive, time consuming, possibly dangerous, and far less versatile.
However, a variety of FEA tools are available to the designer, each with its own strengths. And while careful application is still required to get meaningful results, the tools have become far more accessible to mainstream engineers than ever.
What follows is a look at a diverse range of products and systems developed to survive unusual situations. While many aren't ordinary design problems, their solutions can spawn ideas that find application in more everyday products--the way auto racing improves production cars. Think of them as lessons in survivability.
Bomb-proof ball valve
The ocean presents a uniquely hostile environment for engineered equipment. And the boats that ply beneath her waves, the submarines, are some of the most robustly built and secret of all military vessels.
Fitted to each sub are numerous ball valves used to regulate such critical systems as ballast and trim. Specialty & Ball Valve Engineering (SBVE), Tustin, CA, has supplied such valves to the Navy for more than 25 years, and under several different corporate identities.
Recently, the company applied extensive finite element analysis to design special versions of its DynaflowTM valves for a classified, manned underwater vehicle. The customer asked that the valves not only be as light as possible, but also able to withstand sudden, high-energy pressure spikes without failing.
The source of these spikes? "Submarines can get shot at," says Harry Buehrle, the company's marketing director, "and our valve would see the pulse from the resulting explosion." The ballast tanks are tied directly to the subs' external environment, and any pressure surges would pass like shock waves through the ball valves.
By using FEA, engineers eliminated the need to physically test for the dynamic shock criteria, saving substantial time and money. Computer modeling also helped them trim 4-8 lbs each on the more than 40 valves fitted to the boat.
DynaflowTM ball valves meet the Navy's most stringent criteria, Level 1/Subsafe. Standard models come in five sizes weighing from 12 to 250 lbs, and with orifices from 0.5 to 2.3 inches. Materials include stainless steel, Monel(R), titanium, and Inconel(R).
While designed primarily to handle gases, the valves can regulate fluids as well. All offer zero leakage at up to 6,000 psi and top loading of the internal components for easy maintenance.
Consulting engineer Zach Pursell of Mechanical Analysis & Design Consultants (MADC), Oceanside, CA, performed the finite element modeling of the custom "lightweight" valve using MARC Analysis' (Palo Alto, CA) FEA code. "The biggest questions we had to answer were, would the valve body survive the shock impulse?" he says, "and, if so, what about the seals and internal components?" While weight was also a design driver, the dynamic impulse condition proved to be about five times more important in determining the valve's final configuration.
Pursell modeled each valve part separately and used MARC's adept handling of nonlinearities and contact to analyze interaction between components. "There really is no other code on the market that will model contacting bodies like MARC will," he says.
Limb lopper takes abuse
Ed Danzer understands a thing or two about designing machines to survive rugged environments. His company, Danzco, produces--among other things--the PT-20 series of delimbers for the logging industry that, like a kid popping tops off dandelions, can strip branches from a felled tree in seconds.
The portable machines consist of a heavy steel base with bladed legs that plant into the earth for stability. A small on board diesel engine powers a 1,000-psi hydraulic circuit that drives two opposing semicircular steel knives via 2.5-inch-bore cylinders.
Cut trees are pulled between the knives by a crane-like excavator or tractor. The knives clamp the tree trunk with 500 to 1,000 lbs contact force, trimming the limbs as close to the bark as possible. The trees can range from two to 20 inches in diameter, and Danzer has seen customers delimb up to 250 logs in an hour.
He's also seen them inadvertently tip over a 7,000-plus-lb delimber by using it a little too vigorously. And he's seen the 3/8-inch-thick plate motor mounts bend when an excavator operator picks up and drops a machine in a new location like a child's tinker toy.
But what drove him to using FEA was when users began experiencing bent knives. The two-inch-thick knife arms made of A-36 steel plate measure six inches wide at the shaft. They are designed to take 15,000-20,000 lbs of force. "But to eliminate warranty issues, we started using finite element modeling to figure out what the forces and stresses really were," Danzer says.
He leverages a combination of PC-based software tools: SolidWorks 97 (SolidWorks Corp., Concord, MA) for CAD design; Working Model (Knowledge Revolution, San Mateo, CA) for kinematics; and COSMOS/Works (Structural Research & Analysis Corp., Los Angeles, CA) for FEA. All but six hoses of the delimber's 1,200-component assembly are completely solid modeled. The design pushes the limits of the software and hardware--an Intel Pentium 166-MHz PC and Digital Equipment 433 workstation.
Though supplied by different vendors, the programs work well together. "Instead of having to know how to create geometry in three packages, I only have to know one--SolidWorks," he says. "I can click on motion and throw the model into Working Model and get the forces, and then do the same thing for finite element modeling."
Working Model helps Danzer determine the magnitude and direction of the forces being generated. "One of the toughest parts is knowing what values to put in the FEA and where to place them," he says. With the delimber, forces on the knives actually exceeded 30,000 lbs. By changing the material to stronger A-514 plate, he solved the bending problem without having to alter the knives' geometry.
Not one to rest, Danzer is developing what he says is the industry's first Cut, Fall & Load grapple (CFL). It's a single system that can reach out and grab the tree, cut it off, pull it through the delimber, buck it to length, and load it on the truck--replacing the function of four machines.
Again, the possibility of rough usage is playing a key role in the design, which began prototype testing in May. "If they attach the grapple to a 100,000-lb excavator and start pulling stumps, we've documented pressure spikes in the hydraulic cylinders of 20,000 psi," he says. "The cylinder pins are 2.5-inch, 4140 induction-hardened steel, and I've seen them sheared off." Building the CFL to survive, it seems, is another job for FEA.
Helicopter airbag softens blow
To promote a high-tech image, automobile manufacturers often allude to their use of "space age" technology. But if engineers at Simula (Phoenix, AZ) have their way, military helicopters might soon benefit from an safety system first introduced in the family car: the airbag.
The project stems from an Army Small Business Innovation Research (SBIR) program dating to the early 1990s, but has been expanded to other military and civilian aviation organizations. Its ultimate goal: to develop a cockpit airbag system, including the necessary sensors, logic, firing systems, bag configurations, and packaging, a helicopter might need. Target rotorcraft include the AH-64 Apache, UH-60 Blackhawk, and RAH-66 Comanche.
Helicopter crews should especially benefit from airbags. The cockpits are more snug than automobile interiors and populated by hard, closely positioned consoles and controls that can strike the head and upper torso during a crash. Accidents usually involve large vertical forces. And though a special "stroking" seat significantly reduces spinal injury, it also brings the crew closer to secondary strike hazards. Many crashes involve lateral motion for which the restraint harness offers little protection.
To better understand and predict body kinematics, engineers turned to finite element modeling. "Our main goal was to see if it was possible to use analysis tools to examine how the occupant would react to a deploying airbag in a crash situation," says David Furey, analytical engineer at Simula. "We want to duplicate in the computer all the military-defined crash situations and design an airbag that would protect during them." A second goal: to see if computer simulation could ultimately reduce the number of tests required.
Furey used MacNeal Schwendler's (Los Angeles, CA) MSC DYTRAN for the analysis and PATRAN for pre- and post-processing. He ran it on a Silicon Graphics (Mountain View, CA) Power Challenge with four R8000 CPUs. The computer mannequins were defined by ATB (Articulated Total Body), a public-domain, rigid-body model maintained by Wright Patterson AFB that links well with DYTRAN.
Furey modeled complete cockpit interiors with two occupants and three airbags--front and two side bags--and simulated complete crashes from zero to about 120 msec with a variety of firing times for the bags. Simulated vertical velocities ranged from about 30-42 ft/sec, resulting in as much as 45g peak deceleration for the cockpit.
The airbags were modeled as triangular finite-element membrane elements. Due to space constraints in the cockpit, the bags tend to be unusual shapes, and the biggest challenge was simulating the unfolding action. The problem is compounded by the possibility of a partially deployed airbag striking an out-of-position crew member.
"I have to accurately depict the bag unfolding as it hits the occupant, and that is very difficult to do," says Furey. "DYTRAN was the only code we looked at that could model the complex geometry of the bag as folded and model the contact of the folds as it deployed."
For those things DYTRAN couldn't do Furey turned to MSC. Several times the company made quick changes to the DYTRAN code to accommodate special requests.
Should further tests prove the accuracy of the simulations, an opportunity exists of using them to save money and help accelerate future designs.
Shipping container survives falls
Many times shipping containers have to survive unusual events to protect their cargo, none more so than the new AT400-A. Spawned from a joint Department of Energy project, the cylindrical container--looking roughly like a 30-gal drum--was developed for the transport and storage of nuclear components, such as the plutonium "pit" that forms the heart of an atomic bomb.
Various aspects of the AT400-A's design were distributed among several DOE institutions. The structural development fell to Lawrence Livermore National Laboratory (LLNL), and engineers there made significant use of DYNA 3D, a finite element code developed at the lab that is particularly adept at high-strain-rate, non-linear, large-deformation events.
Specifications called for the container and its contents to survive a 30-ft fall onto a hard surface. "The anticipated accidents are with ground transport," says Peter Raboin, methods development group leader at LLNL. "We had to design it to absorb a certain quantity of energy over a certain displacement."
The container's outer layer is designed to mitigate fire. Inside are structural elements for impact and shock. Using DYNA 3D, engineers designed a suspension structure towards one end of the can consisting of a "C" channel running around the circumference of the container. Suspended from the flange is a fixture, like a chuck, that holds the nuclear component.
The "C" channel was designed to distort and absorb energy during an impact. "If it falls on one end of the can the flange compresses, and if it falls on the other it extends," Raboin explains.
Less simply, engineers purposely added sharp corners to the flanges' attachment bolt holes to actually promote tearing of the metal. "This nearly doubled the energy that the flange alone could absorb," Raboin adds. Lastly, to fine tune the structure for the desired deceleration values, the engineers created special shims.
The extremely complex DYNA 3D models contained about 70,000 nodes and 27 contact surfaces. They took as much as 140 hours to run on a Cray T3D. "It was frustrating." states Raboin. "The analysts couldn't keep up with the drafting designers."
Part way through the program, however, engineers gained access to the Lab's Meiko (San Francisco, CA) CS-2 massively parallel processing (MPP) computer. As a result, calculations took less than 10 hours, and Raboin notes that they gained the capability of performing full systems analysis rather than just individual components.
In the race against the drafters, the analysts caught up and actually pulled ahead. Says Raboin, "It was the first project I'd been involved in where the analysis had an impact on the design before it was finished."
Portable scanners take a beating
Mitch Maiman has heard about users of his company's barcode scanners who pay so little attention to the product that they casually dangle them off the side of a moving forklift, banging them into objects while driving along. That's exactly how he likes it.
"What differentiates us from our competitors is our ability to develop a rugged product that can take the shocks and falls that are part of our customers' world," he explains. "They should be able to use it without worrying about dropping it."
Maiman is the Senior Director of Scanner Product Engineering for Symbol Technologies (Holtsville, NY), a company that manufactures portable data-capture terminals, wireless data communications equipment, and barcode scanners. These ubiquitous devices are used daily by express-package delivery personnel, rental-car agents, and warehouse inventory checkers.
At the heart of most of these products (and many others) is Symbol's SE-1200 family of scan engines. Though comprised of seemingly delicate lasers, oscillating mirrors, and electronics, they'll withstand 2,000g shock pulses.
To ensure the products survive, Symbol engineers make extensive use of finite element analysis. Product definition is done with Structural Dynamics Research Corp. (Milford, OH) IDEAS software and finite-element analysis with ANSYS from ANSYS Inc. (Houston, PA).
SE-1200s generate a rapidly scanning line of light that, when pointed at a bar code, reflects back into the scanner to be interpreted. The scanning is generated by a patented mechanism that reflects a laser off an oscillating mirror. The mirror and a magnet are attached to a Mylar spring and driven at the assembly's resonance point. "A big use of the analysis is for the fatigue of the motor, which sees millions of oscillations, and for determining the natural frequency," says Maiman.
But shock analysis is an even bigger design driver. An example is the PPT 4600 series of portable pen terminals. Engineers were challenged to make the devices withstand repeated 2m falls to concrete. They also weigh just 1 kg and must remain affordable--ruling out exotic armor.
Compounding the problem, each terminal contains a glass LCD touch panel for which, Maiman notes, the manufacturers could supply virtually no mechanical data. "We had to reverse-engineer them," he says, "and characterize the displays ourselves."
These characterizations resulted in detailed FEA models of the LCDs used to accurately simulate real-world mechanical performance. Leveraging these tools, engineers designed a patent-pending frame within a frame to protect the display. An internal frame supports the LCD; it, in turn, is attached to an external frame formed of injection-molded plastic overmolded with rubber. "The computer modeling let us engineer the connection between the inner and outer frames to get the level of energy absorption we needed," Maiman explains.
Such attention to detail pays off in the end. "It's somewhat expensive to analyze everything; it costs money up front," says Maiman.
Cyber contacts
You can reach the following companies mentioned in this feature on the Internet. Please tell them that you were referred by Design News.
ANSYS: www.ansys.com
Lawrence Livermore National Laboratory: www.llnl.gov/
ESI North America: www.esi.fr/
MacNeal Schwendler: www.macsch.com
MARC Anaylsis: www.marc.com
Structural Research & Analysis Corp: www.cosmosm.com
Symbol Technologies: www.symbol.com
McDonnell Douglas Corp: www.mdc.com
SolidWorks Corp: www.solidworks.com
About the Author(s)
You May Also Like