October 20, 1997
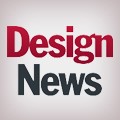
Gainesville, FL--While space station Mir has demonstrated sustained human space habitation, it has also revealed shortcomings of current oxygen-generating technologies for manned interplanetary exploration. Maintaining the required supply of chemical reagents and adsorbent materials or membranes may prove impractical at locations far from Earth. That's why NASA's Advanced Life Support Program is funding research at the University of Florida. A novel mechanical gas separation technique, developed here, may remove CO2 from air with rapid tube oscillation, eliminating the need for chemical supplies.
As part of a masters thesis, Mark Poplasky, graduate student, and Brandon Crews, research assistant, work under professor of chemical engineering Dr. Ranga Narayanan's guidance. The focus is on removing carbon dioxide (CO2) or ammonia from a species-air mixture, providing revitalized air for reuse. The team has three goals:
Verifying gas separation by pulsating flows applied to CO2
Experimentally determining optimum operating conditions
Advancing the theory of gas dispersion by means of pulsating flow fields.
The basic principle of employing oscillating flows to enhance separation in gas mixtures has already been tested. "We intend to modify the theory, model it experimentally, and substantially increase species removal," says Narayanan. To meet its goals, the team began designing a device to demonstrate gas separation with oscillatory flow. In the process, they ran into an unexpected roadblock: locating a linear bearing to support the machine's oscillating plates. Although the loads were not particularly high, finding a bearing capable of handling linear speeds up to 22 ft/sec and frequencies up to 10 Hz proved to be a challenge. "Most linear bearings only handle velocities of 10 ft/sec," notes Poplasky. That's when Port Washington, NY-based Thomson Industries Inc. answered the call.
In an effort to find new ways to remove CO2 from air, NASA's advanced life support program funds University of Florida's development of oscillatory separation. 22-ft/sec linear velocities at 4.5-Hz frequencies would cause most linear bearings to come apart. Thomson Industries Inc.'s RoundWay roller bearing supports the separator's oscillating plate.
Old rugged bearing. After the students contacted Thomson's Chris Stabile and presented the application's requirements, Stabile knew the only linear bearing on the market capable of surviving in this application was Thomson's RoundWay roller bearing, even though it has been around since the 1960s. Stabile convinced Thomson to donate its products for the research effort. "This project never would have got off the ground without them," explains Narayanan.
What is unique about the RoundWay linear bearing is that it uses concave rollers, which run around a solid core, and are held in position by a chain--something like a tractor tread. The chain maintains roller orientation and spacing at acceleration rates as high as 137m/sec2, well exceeding the application's requirements. Stabile traveled to the application site at the university's Bifurcation and Non-Linear Instability Laboratory earlier this year to inspect the bearings. "After two years in operation, the bearings still perform perfectly," he reports.
In the experimental separator, RoundWay bearings support the oscillating plates used to drive the concentric tubes. The tubes connect the two finite reservoirs, to which a gas mixture and carrier are continuously fed. Two 1-hp, 220V, 3-phase vector motors from Fort Smith, AR-based Baldor Electric Co. drive two 80-lb flywheels at speeds up to 270 rpm. A sliding bearing converts the flywheel's rotary motion into the sinusoidal oscillation plates that drive the tubes.
Tubes may move either in-phase or out-of-phase with each other. Oscillating the concentric tubes in phase significantly enhances mass transport. To avoid direct mixing between the two reservoirs, oscillation amplitude is controlled to something less than 40% of the pipe length. Two recirculation fans keep each reservoir well mixed.
For air revitalization, a gas mixture of CO2 and air, denoted Q0 in the experimental setup figure, is continuously fed to one reservoir. Water vapor, Q3, is fed to the other reservoir. Water vapor, the carrier gas, is a good transporting agent for CO2 separation for two reasons:
CO2 transports preferentially into water vapor because of its diffusion coefficient compared to that of air.
Water vapor easily condenses out of the gas streams.
The carrier gas also helps control the separation. Adjusting carrier-gas counter flow controls the concentration gradient, the driving force behind diffusion. Bulk convective flow manifests as a net flow, Q4, inside the tubes. By altering Q0 and Q3 input streams, flow Q4 is adjusted from zero on up. The net effect of increasing carrier gas counter flow is a larger concentration gradient and increased separation efficiency. The trade-off is a decrease in rate of mass transfer. It seems counter-intuitive, but no bulk flow is necessary to ensure separation, according to Narayanan.
While diffusive flux alone remains a constant, convective gas flow generated by tube oscillation gives engineers the ability to enhance mass transport, according to Narayanan. Pipe dimensions, oscillation frequency, and relative diffusivities of each component, all determine the rate that each component is transferred. Other factors that can influence mass transport in this separator design include: a temperature gradient between the two reservoirs, and buoyancy-driven convection. Although more analysis is needed in these other areas, the net result from oscillation alone is that gas enriched in air, Q1, issues from the outlet of one reservoir, and gas enriched in CO2, Q2, flows from the other. Carrier-gas removal from each enriched outlet flow, and a multiple-stage design could achieve more complete separation.
Test & measurement. The system uses mass flow meters from Andover, MA-based MKS Instruments Inc. to measure all flow rates. A Hewlett- Packard G-C measures oxygen concentration. For a three-component system composed of O2, N2, and CO2, it is sufficient to measure total density and oxygen concentration in order to determine individual species concentration, according to Narayana.
The overall arrangement maintains the concentrations of species, and will establish a time-averaged steady concentration gradient along the tubes. It's the time periodic hydrostatic pressure gradient applied to the gases that actually establishes oscillatory flow, according to Narayana. The flow, and the associated concentration distribution reach a "steady time-periodic" state several cycles after oscillation starts. This means that the flow and concentration fields are still a function of time, but they repeat periodically without any change between cycles.
The inner tube is plugged so that all transport takes place in the annular region between tube walls. Using concentric tubes, instead of a single oscillating tube, increases the tube-wall surface contact for the gas. "With more tube wall, there is more boundary area for the molecules to diffuse to and from," Narayana notes.
During the first part of the tube's oscillation cycle, flow is from left to right. A spike from the viscous shear between the tube wall and gas forces fluid particles near the pipe wall to remain at rest. As a result, the interface between the gas mixture and pure gas is elongated and its surface area enlarged. Diffusion of CO2 now occurs not only in the axial direction, but, more importantly, in the radial direction from the core into the boundary. Radial diffusion is promoted by two things:
The interface area being larger than the pipes' cross section
Greater concentration gradient between the core and the region close to the pipe wall than the initial axial concentration gradient.
A number of CO2 molecules are transported from the core to the boundary through this radial diffusion. At the moment the convective flow is reversed, the diffusion situation is also reversed since the radial concentration gradient changes its direction. Molecules that have just diffused radially will diffuse back into the pipe's center where the carrier fluid comes from the right hand side. When the flow comes again from the left, the core-to-wall diffusion also comes with fresh CO2 from the left. CO2 molecules transported in the previous cycle continue moving to the right with each cycle in a zig-zag fashion.
As the flow goes back and forth, the core-to-wall-to-core procedure repeats itself. The result: CO2 molecules transport from left to right with many steps of stopping at the boundary, then reboarding on the core flow. This transforms the axially reciprocating convectional motion into a unidirectional mass transfer of CO2 from left to right.
Timing is everything. Oscillation timing plays a key role in dispersion of pulsating flows. The time periods allowed for the two processes--axial convective motion of the fluid and the radial diffusion of the molecules--have to match each other for optimized time-averaged unidirectional mass transfer.
If axial motion is too fast, radial diffusion slows down because only a small number of CO2 molecules have time to diffuse between the boundary and core region. On the other hand, a small overall axial mass transfer occurs when the axial motion is too slow compared to the radial diffusion speed. Reason: CO2 molecules must wait at the boundary until the radial concentration gradient, established by the axial convective flow, is large enough for diffusion.
Maximum mass transfer occurs when the radial diffusion time from tube axis to its wall is approximately equal to half of the oscillation period. An optimum frequency is tuned, in fact, there is a different characteristic frequency depending on what species is being removed.
Another unknown, according to Narayanan, is the effect of turbulence in the separator tube. "We don't fully understand how turbulence affects mass transport, all we know is that it will blur the theoretical results." That's why maximum frequency is held to 4.5 Hz.
Although the fundamental physics of enhanced removal depends on the molecular diffusivity of CO2 relative to the diffusivities of oxygen and nitrogen, it's the pulsating flow in conjunction with these relative diffusivities that enable enhanced removal.
One way the space program could explore separator's potential is in closed capsule air revitalization systems. In such an application, the CO2-air mixture from the crew's quarters would be divided into two streams. One stream, rich in CO2, could be processed in a bioconversion unit in a closed-loop scheme, or expelled in an open loop. The other stream would be returned as breathable air.
This type of separator might also be adapted to remove other organic contaminants from air, such as build up from solvent spill, or from internal spacecraft materials gassing off. It should be noted that although data proves this device enhances mass transport, no data on gas separation is available. Clearly, before this type of mechanical separator blasts into orbit, more work must be done.
In the next year the team will design and build another device that uses bellows on each chamber to induce pressure-driven flows rather than moving-tube walls. One advantage might be reduced power consumption.
Other Applications
Particle classification
Separation of weak and strong electrolytes
Removing organic contaminants from air
How oscillatory separation works
Oscillatory separation is a mechanical separation technique under study at the University of Florida. It involves gas separation by enhanced diffusion in oscillatory flows. Because no chemicals, adsorbents or membranes are used, it may provide an alternative air purification method for NASA's Advanced Life Support Program.
A chamber on the left end of tube contains CO2 and air. The right hand chamber would contain a carrier such as water vapor. Consider the mass transport at any point in an oscillating pipe that connects the two chambers.
A.) CO2 molecules move from left to right under the influence of normal diffusion.
B.) Induced by tube oscillation, the gas begins oscillating rapidly from left to right. CO2 molecules diffuse radially into the boundary region near the tube surface, as well as axially as in normal diffusion.
C.) As the tube stops and begins to reverse direction, CO2 molecules have moved a net distance.
D.) When tube motion reverses and begins moving to the left, CO2 molecules radially diffuse once again back to the core region of the tube.
As the fluid moves, the CO2 transports in a zig-zag fashion through the tube to the right end. Although O2 and N2 molecules in air move in a similar fashion, they move at slower rates than CO2. Result: a separation between CO2 and air takes effect. The carrier, enriched in CO2, is then recovered by condensing the water vapor carrier.
Additional details...Contact Dr. Ranga Narayana, Chemical Engineering Dept., University of Florida, Gainesville, FL 32611, E-mail: [email protected]
Experimental Oscillatory Flow Data |
Outer Tube (Hz) |
1 |
1 |
1 |
2 |
1 |
1 |
2 |
2 |
*UNITS: 10-6 cm3/sec (NORMALIZED) |
About the Author(s)
You May Also Like