Cool Screws
July 19, 2004
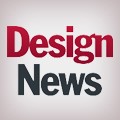
|
Ball screws can transmit several kilowatts of power. This doesn't sound like a lot, and it is insignificant compared to the power consumption of a linear motor. Most of the heating in a ball screw drive occurs in the servo motor, where heat dissipation is easy to deal with and doesn't cause major problems. Thanks to their high efficiency, only a fraction of the drive power (typically less than 15 percent) is lost in ball screws.
But with machines getting faster, even this fraction can no longer be ignored. The heat generated has an impact on accuracy not only in the ball screw but can migrate into the surrounding structure. In physical effects, for example, steel in the ball screw shaft expands around 12 microns/m/ degrees C leading to greater position shifting the larger ball screw.
Here's how to calculate what to expect and how to deal with it:
Basic Ball Screw Physics
Efficiency is defined as power output per power input. In mechanical power transmission elements-i.e. ball screws-the difference comes from friction between moving parts. In a ball screw it's mainly friction of the balls rolling in races between the shaft and nut.
Ideal rolling of a ball on a surface with less curvature than the ball causes no friction since the ball contacts the track surface in an infinitely small point. In an infinitely small contact point there is no relative motion between contact surfaces, so there is no work in the physical sense (force times travel).
But in the real world there is a contact patch with finite dimensions. Patch size causes "forced slip" between ball and surface as the ball moves along the track. In the same way, differential speeds in the contact patches of a shaft-to-ball and ball-to-nut cause slip. Both combined are responsible for the coefficient of rolling friction in ball nuts.
A coefficient of friction is expressed as friction force parallel to the direction of motion, divided by the force perpendicular to it. A close enough approximation for the coefficient of rolling friction in a ball nut is 0.008 for any nut having two-point contact on the balls, and 0.01 for any nut having four-point contact.
The friction angle n is defined as the arctangent of the coefficient.
Now suppose a force pulls on the screw, tangential to its surface and parallel to the ball track. To convert this force to a moment, a radius is needed. The ball center diameter works best, although it's not exactly correct. But it is close enough and, because the ball center diameter is normally identical to the nominal diameter, they can be interchanged.
a=arctan(h/o- dN)
where,
a: Helix angle
h: Lead (distance track "moves" along screw in one turn)
dN: Nominal diameter
Now,
Mr=Fpr- rn- tann/cosa
n: Friction angle
Mr: Friction torque
Fpr: Preload force
rn: Nominal diameter/2
That equation can be used to determine the preload torque of a ball screw. Then multiplying by the rotational speed gives power,
P=M- u
where,
P: Power
M: Torque
u: Rotational speed
The power equation determines power consumption if the ball screw runs with no outside load.
Once an outside thrust is present, we have to use some more involved math, namely:
c=tana/tan(a+n)=efficiency
This efficiency is used to determine how much additional power is lost to friction when the ball screw produces outside thrust. It does not include the preload torque.
The analysis has two components: One is the moment that is due to preload (and completely independent of outside thrust) times speed, and the second is a usage factor that we must apply to the input power. Output power can be calculated from the duty cycle:
P=F- v
where,
F: Force
v: Velocity
Identify the Heat Load
Average output power divided by the efficiency is input power, and the difference between powers is the loss that heats the ball screw. Preload torque times mean speed must be added to the loss. A usage factor (duty cycle) must be applied to reflect the ball screw dwell times (stopped conditions) that are not included in the duty cycle.
In order to go from heating (power) to temperature rise, the rate of heat dissipation is needed. But due to the variety of factors, it is almost impossible to approach the problem from an analytical standpoint. Instead it is necessary to use data obtained from actual applications as a guideline for the expected temperature range.
The result is an estimated delta of the ball screw temperature over ambient that depends mostly on the power loss in the ball screw. Unusually strong heating from support bearings, poor heat dissipation due to a small, confined space around the screw with no air exchange, or other factors may cause significant departures from these estimated temperatures.
Take Steps to Reduce Heat
If the resulting temperatures are too high the first step is to see if the heat generation can be minimized. Heat drivers are the diameter, speed (in rpm), and lod.
Reducing the diameter is critical, because the reduced cross-section would also cause lower axial stiffness and lower load capacity. Increasing load to reduce rpm is a very efficient method, provided sufficient drive torque and encoder resolution are available. Finally, if payload is reduced, some stiffness will be sacrificed.
If there is an issue with unloaded balls (no preload leading to ball impacts with each other or binding), single nuts with four-point ball contact can be used instead of double nuts with two-point contact. There is no unloading of balls in single nuts and preload can be relatively low-a smart alternative because it increases fatigue life and saves money.
The effects of elevated screw temperatures on accuracy can be offset by pre-tensioning using thrust bearings on either end of the ball screw. By means of shims or an additional locknut, the screw can be put under tension when cold. An increase in temperature and the resulting thermal expansion will then just reduce tension, but not cause the screw to grow in length.
But this method requires heavy thrust bearings, which in turn contribute to heat generation. Because the elongation of the screw through pretensioning must be greater or equal to the thermal expansion at the anticipated maximum temperature, the worst-case scenario dictates the pre-tension forces. As a result, if the heating isn't the worst case, the screw runs colder and leaves the thrust bearings with significant load that might reduce their life. Pretensioning is only practical when the anticipated temperature rise is not too large.
Add Forced Cooling If Needed
If all this fails, forced cooling is available. This is usually accomplished in two ways: Hollow shafts with internal cooling, or a nut with a liquid-cooled jacket. Hollow shafts are the more common method. They are generally troublesome when the shaft is rotating because the necessary rotating unions are expensive and often need maintenance; hollow shaft cooling is easier when the nut rotates instead.
A separate shaft cooling loop has the advantage that the liquid is clean and doesn't carry any abrasive debris to cause wear on moving parts such as seals. But a separate system costs money and needs to be monitored. A loss of fluid cuts efficiency and can cause excessive temperatures of the ball screw shaft, which in turn might damage support bearings.
An alternative is to use cutting fluid in CNC applications. Such fluid is always available and will be replenished before it runs out-unless the machine is used for dry machining. Despite filter screens, abrasive particles will cause damage to the moving parts with time.
Cooling the shaft addresses its thermal expansion and also keeps the temperature in the immediate surroundings of the screw lower. Shaft cooling does not address the issue of heat dissipation from the nut into the slide. For stiffness, the nut is connected, via a relatively large surface, to the slide in a metallic contact with good thermal conductivity. If the nut gets very hot, the heat dissipates efficiently into the slide. Insulating the nut isn't practical because the heat will eventually flow into the slide-so the nut must be cooled.
Care is the ultimate byword. As both shaft and nut expand, the preload will be mostly unaffected. If the shaft heats up and the nut doesn't, the preload goes through the ceiling quickly. So it's imperative to monitor the cooling system and issue a warning or even shut down the machine if temperature control is no longer assured.
About the Author(s)
You May Also Like